GM crops: Part 3 transgenic biotechnology
In this four-part series, Kevin Folta, Ph.D., examines the development and uses of high-performance plants through the lens of science.
October 10, 2012
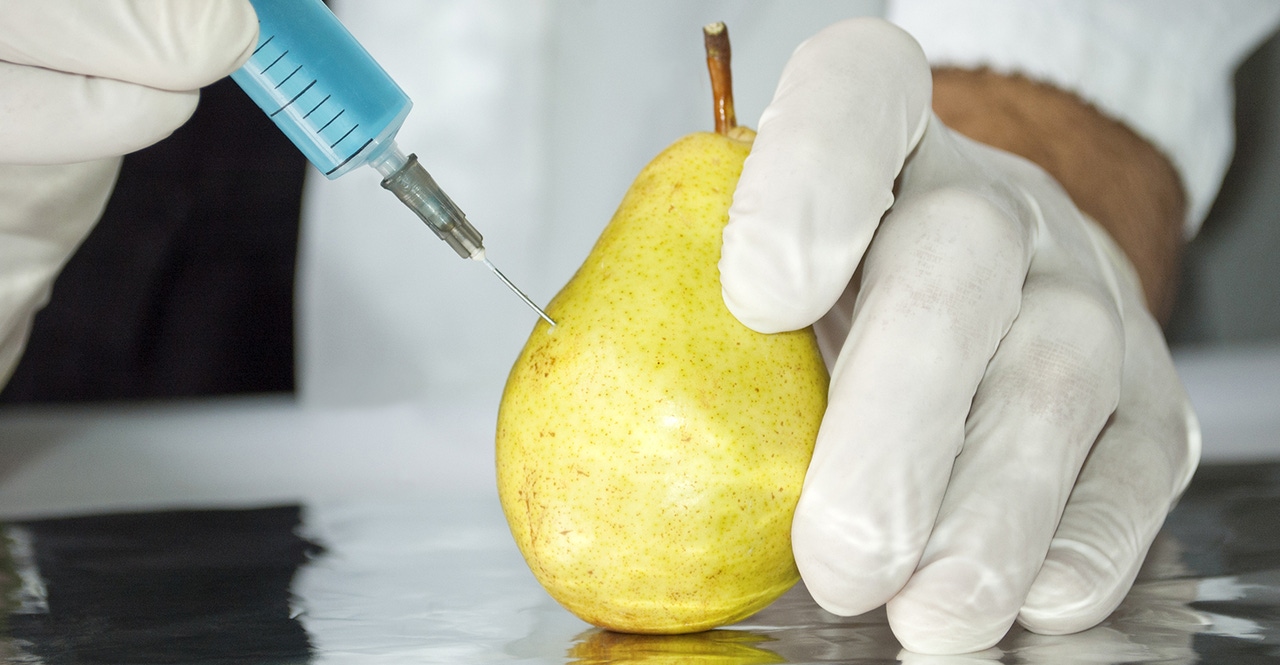
Todays genetic engineering processes, especially those used on food crops, are the subject of much contentious debate. Much of that debate centers on the assertion that the process is unnatural" and therefore the result of the technology is inherently dangerous. Opponents use frightening images of Frankenfoods" and assert natural" heirloom varieties are the only plants man was meant to eat. In this four-part series, Kevin Folta, Ph.D., examines the development and uses of high-performance plants through the lens of science.
Transgenic biotechnology
As the 1980s progressed, so did a new technologythe ability to transfer a single gene of biological importance to a new plant. Transgenic technology and the larger practice of biotechnology were born. Plant scientists all over the world reveled at the potential power of the ability to move single genes into a given genetic background. At the time, plant gene discovery and isolation was a slow and difficult process. Still, they imagined a time when genes for disease resistance, higher yields, resistance to cold, heat, drought and a multitude of other stresses might help the American farmer. Many thought of ways in which it could change where plants were grown, produce more food with less input (fertilizer, pesticides and labor), and perhaps even make higher-quality designer food. The ability to help those confined to areas replete with poor soils, disease pressure and low nutrition was an obvious priority.
Transgenic technology (also commonly known as genetic engineering or genetic modification) has been with us ever since. Most transgenic plants made are for research purposes only. In plant biology weve learned a lot about the inventory of plant genes, especially as the number of fully sequenced genomes continues to increase. However, a sequenced genome is only a parts list, and science has no idea what the majority of those parts do. Transgenic technology gives us the tools to test what any given gene does by turning it on all the time, or by suppressing its expression. These tests of loss- and gain-of-function are the gold standard for learning about what genes do. Almost everything we know about gene function has been tested and confirmed (or sometimes refuted) using transgenic technology. These products are almost never commercialized, but they provide amazing information that helps us understand genes and plant biology.
Practical application for transgenic biotechnology
Clearly transgenic technologies have been of tremendous value to basic plant science, but what about practical application? In some cases, the tests in transgenic plants guided efforts in traditional breeding. While plant breeding has a great capacity to move a desired trait, it brings along substantial undesirable genetic real estate. Removing the junk that travels with the good stuff requires time, labor and, if you are working a crop like apples, millions of dollars to grow and maintain acres of trees for years. However, once a genes function is known, we can move it by cross-pollination and then verify its presence using molecular markers and other biotech tools. Today, marker-assisted selection hastens the arduous process of plant breeding.
But how about a faster track? What if a breeder could move that one gene, controlling one trait? This has been possible for over 25 years, and for the last 15 has been widely adopted in the United States. Today, transgenic plants represent over 90% of acreage of corn, soy, canola and cotton, and are present in 70% of grocery store items.
Today, two genes are widely implemented in transgenic crops. The first confers resistance to the herbicide glyphosate, and are commonly known as. Roundup- ready crops. Glyphosate, the active ingredient in Roundup herbicide, targets 5-enolpyruvylsikimate-3-phosphate synthase (EPSPS), an enzyme required for synthesis of certain amino acids (remember that enzymes are proteins that facilitate efficiency in chemical reactions). Glyphosate binds this enzyme with lock-and-key specificity, interrupting synthesis of critical proteins. Plants treated with glyphosate die from this block.
However, a bacterial form of the same EPSPS enzyme has a minor difference. A single amino acid called alanine is substituted for glycine in the EPSPS protein. The change is extremely minor, yet disables the enzymes ability to bind glyphosate. No binding, no inhibition, no sensitivity to the compound. So, what if the enzyme that does not bind glyphosate is introduced into plants?
The bacterial form of the enzyme was transferred to plants. The plants now have a form of the same exact enzyme that has an identical role in metabolismbut it cannot be inactivated by glyphosate. Plants containing the new slightly-modified version of the same enzyme grow well in the presence of the herbicide, where non-transgenic plants around it yellow and die.
To take advantage of this trait, farmers place glyphosate-resistant seeds into soil and allow them to germinate, along with the weeds that naturally occur. Shortly thereafter, the fields are sprayed with glyphosate, killing the weeds and leaving only the crop plants, which can then outcompete weeds for water, nutrients and sunlight.
Benefits and drawbacks of transgenic biotechnology
The technology has environmental benefits and drawbacks. It saves farmers money, fuel and labor. It allows farmers to till less, preserving valuable topsoil. The environment benefits, because herbicides with worse environmental impact and animal/human toxicity have been shelved. The downside is that, after 15 years of use, some weeds are gaining resistance to the herbicide, requiring higher rates of application and, in some cases, usurping its utility altogether. These herbicide-resistant weeds now require improved alternative solutions, such as other herbicides, to remove them.
The other major gene introduced to plants is called Bt, short for Bacillus thruengensis, a bacterial species that makes a small protein that is toxic to certain beetle, moth and butterfly larvae. These larvae can cause massive damage to various crops, especially corn and cotton. Bt has been used for decades in organic crop production because it naturally suppresses insect damage imparted by feeding insect larvae. Bt has been studied since the 1920s for effects on animals, as well as the crop itself and has always been considered a safe alternative to synthetic, broad-spectrum insecticides.
The Bt protein is powerful because of its mechanism of action and specificity. When larvae consume the Bt protein, a specific enzyme in the bugs gut converts it to an active form. The active protein then binds a receptor in the wall of the gut, where it forms a pore, causing leakage and death of the gut cells. The larva eventually dies. The protein is processed only in the larval gut and binds only the gut receptors of specific insects. These attributes make it harmless to other animals, including humans.
Developing corn cobs are succulent targets for insect invasion, such as corn earworms and corn borers, whose larvae feed on the ear, damaging it and rendering it unsalable. To combat these problems, farmers relied on fuel-guzzling spray planes to douse fields in broad-spectrum insecticides at ear set.
With its extensive record of safe usage and discrete insect targets, the Bt gene (called Cry1A) was introduced into corn. The corn plant expresses the Bt protein, and upon the first nibbles the larvae die, sparing the crop. No spray planes necessary. According to the National Academies of Science, this technology has cut insecticide use by 70% to 80% for corn and cotton. There are no widely scientifically accepted downsides to Bt usage when used as prescribed.
Kevin M. Folta, Ph.D, is an Associate Professor in the Horticultural Sciences Department, and Graduate Coordinator for the Plant Molecular and Cellular Biology program at University of Florida. He was the contributing author of the sequence to the strawberry genome and studies how genes and the environment work together to improve plant traits. He also has been recognized for his commitment to community outreach and student training in laboratory science. Contact him at [email protected] .
You May Also Like